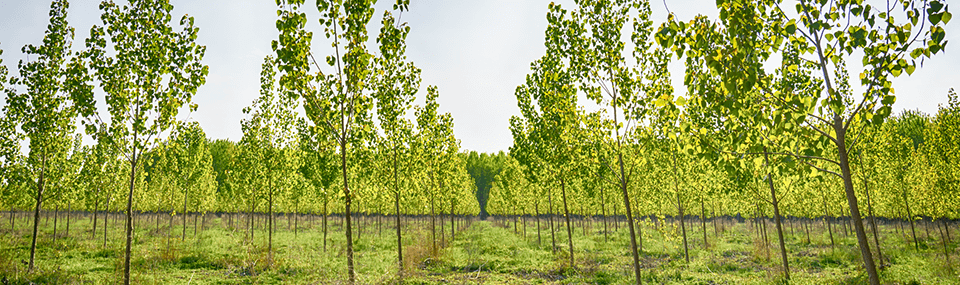
The math is clear—meeting Paris Agreement temperature goals will require not just reducing emissions but also removing them from the atmosphere. Studies suggest that by 2030 as much as 6 gigatonnes of carbon emissions needs to be captured and permanently stored each year—a target feasible with philanthropic grantmaking to catalyze investment.
Forthcoming International Panel on Climate Change (IPCC) scenarios indicate that staying even within the agreement’s less ambitious limit (2 degrees Celsius) will require gigatonne-scale carbon dioxide removal (CDR). Figure 1 displays removal ranges by year, based on older IPCC scenarios that rely in part on mitigation, technological breakthroughs and other assumptions. To update older studies, ClimateWorks Foundation in 2017 convened a few climate modelers to preview scenarios for staying within the Paris Agreement’s most ambitious global warming limit (1.5 degrees Celsius above preindustrial levels). As we discuss in greater detail below, the scale of removal required in the latter half of the century can be reduced with technological breakthroughs as well as with behavioral changes. These findings are timely because ClimateWorks has been working with funders and experts, such as the Center for Carbon Removal (CCR) to explore natural and technological CDR efforts and the opportunities and challenges of deploying them on the required scale.
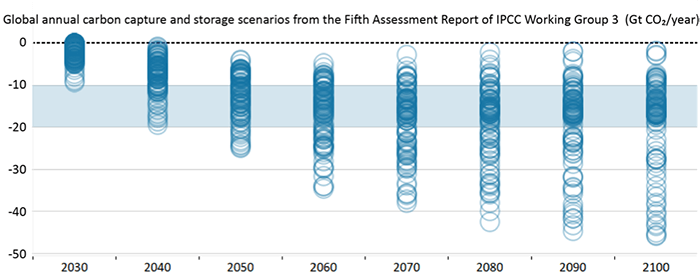
By natural carbon removal, we mean harnessing processes by which the planet already stores carbon dioxide (CO2), for instance, planting forests (afforestation) and adopting no-till and regenerative agriculture practices to help soil increase its carbon storage (Figure 2). Technological carbon removal refers to using sorbents to filter, trap, and use or permanently store CO2. Although many still view these two removal approaches as distinct, we find particular promise in strategies that straddle them, for instance, replacing carbon-intensive steel with wood in construction or harnessing cement that substitutes CO2 for water during the curing process. Tall wood buildings have the added benefit of revitalizing hard-hit timber communities in regions such as the Pacific Northwest.
Common Misconceptions about Carbon Dioxide Removal
- Removal is not geoengineering, which reduces warming (for example, by brightening clouds) but does not reduce the amount of carbon in the atmosphere. Removal reduces carbon emissions from the atmosphere by capturing and reusing or permanently storing them.
- Removal also is not solely about bioenergy carbon capture and sequestration (BECCS), that is, growing trees to combust them for electricity generation and then storing the resulting CO2. Because BECCS serves as a modeling stand-in for all CDR approaches, some assume that removal simply implies afforestation on a scale that would compete with land for food.
- Removal is not just about natural solutions. Because both natural and technological approaches have tradeoffs and are insufficient on their own, both need to be pursued on large scales.
- Finally, some say that removal poses a “moral hazard” to mitigation. But the point is that both mitigation and removal now are required.
Carbon Removal Must Find a Way to Scale Up
Natural solutions such as increasing soil carbon are ready to deploy and low cost, about $10 per ton of CO2, whereas technological solutions such as direct air capture (DAC) are still in the pilot-testing phase and must scale up for their costs per ton of CO2 to fall. Some CDR approaches remain largely within the realm of basic academic research. One of these is weatherization, which uses carbon-absorbing rocks.
CDR approaches—whatever their phase of development or deployment—provide new opportunities to engage non-traditional climate allies—for example, those interested in turning waste CO2 into valuable resources. The ten recently announced NRG-Cosia Carbon X Prize finalists are converting CO2 into a wide variety of products ranging from enhanced concrete to liquid fuels, plastics, and carbon fiber. Such removal approaches provide new opportunities for solutions in economic sectors that can reap co-benefits. In farming and ranching, for example, practices that regenerate soil’s carbon storage capacity can also result in bigger crop yields, healthier forage and livestock, and lower fertilizer and water costs.
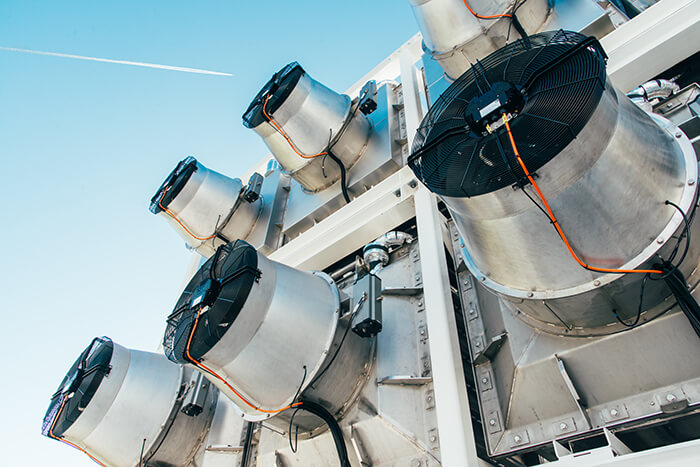
Scalability and Geographic Potential Are Large
All the CDR solutions analyzed in a scoping study we commissioned from CCR have the potential to sequester significant (gigatonne) amounts of CO2 per year. Technological solutions such as DACS and accelerated CO2 mineralization are essentially unlimited in their scalability potential. BECCS is likely to be constrained by land, water, and nutrient use competition. Natural solutions also have large scalability potential, though research is needed to understand their economic potential and tradeoffs as climate change stresses ecosystems and working lands. To better understand how to lessen the land footprint of CDR ClimateWorks commissioned two studies. Colleagues at the Grantham Institute, in a forthcoming study, find that use of breakthrough technologies such as the hyper-loop and hydrogen-fueled aircrafts and enhanced electrification of heavy-duty transportation fleets would reduce overreliance on BECCS by capturing up to 140 gigatonnes of atmospheric carbon between 2016 and 2100.[footnote tooltip=”T. Napp et al., “The Role of Advanced Technologies and Energy Demand Reductions in Achieving Ambitious Carbon Budgets,” Applied Energy (forthcoming). “][/footnote] On the consumer side, energy demand reduction through consumer behavior change and lifestyle change indicate that we could reduce the need to lean heavily on natural solutions later in the century.
Variability in Geography and in Cost
As the foregoing suggests, CDR solutions have wide geographic applicability. Afforestation and reforestation will likely have greater scalability potential in the tropics, where newly established forests sequester carbon most quickly. Technological solutions will likely have greater scalability potential in non-OECD countries. But the upfront public and private investment cost likely will be borne in OECD countries, given their robust innovation and research infrastructure funding mechanisms. CDR approaches also are likely to have different costs at maturity. On the whole, natural solutions are expected to cost less than technological solutions. However, intense competition for land and other resources and changes in climate could make natural solutions more costly, whereas aggressive innovation funding could make technological solutions less costly. Similarly, co-benefits beyond carbon sequestration will affect each solution’s commercial scalability. In general, natural solutions have greater economic and environmental co-benefits than technological solutions.
Four Barriers to Commercialization
CDR solutions are stymied by public and policy makers’ lack of awareness. Another barrier is lack of widely acceptable and cost-effective accounting, measurement, and verification tools and regulations that enable apples-to-apples comparisons across solution categories. Critically, demand for CDR solutions is low in the absence of market incentives for carbon sequestration and of policy and regulatory structures to drive technology innovation and implementation.
Given their deployment readiness, co-benefits, and political acceptability, natural CDR solutions such as increasing carbon storage in soil through regenerative grazing, for instance, are likely to gain the greatest near-term traction. But can these solutions alone be scaled up to meet the 2-degree Celsius goal? Without a definitive answer to that question, we need to simultaneously ensure that technological CDR solutions such as direct air capture (DAC) can be widely deployed within a few decades. That means finding ways to drive down the cost of DAC pilot projects.
Philanthropic Opportunities
ClimateWorks Foundation, in partnership with several of its endowed funders and aligned funders and experts such as CCR, is exploring how philanthropy might significantly advance CDR approaches through traditional grantmaking to advance communications, advocacy, and enabling policies as well as through provision of analytic work that supports finance and market mechanisms to achieve the required gigatonne-scale of those approaches. Look for updates on those efforts in the coming weeks and months.